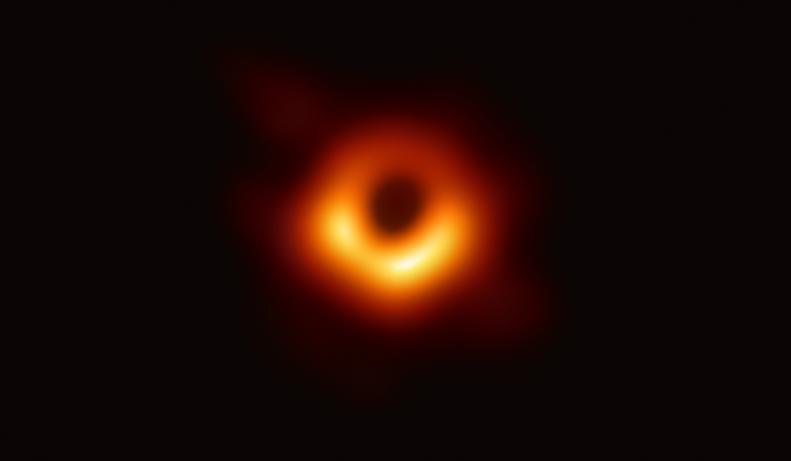
First Image of a Black Hole. © EHT Collaboration.
Investigations into how the black hole went from a paradoxical result of general relativity to an observed reality have been conducted by several research projects at the MPIWG, including "The Renaissance of General Relativity in the Post-World War II Period"—initiated in 2014 by Department I—and by projects within the Research Group Historical Epistemology of the Final Theory Program and the Research Program History of the Max Planck Society.
In Search of Applications: General Relativity and the Collapse of Massive Stars
In the years following Einstein’s construction of general relativity, it was a theory in search of applications: its empirical implications were few, simple, and already worked out. The status of general relativity began to change in the mid-1950s, a period the physicist Clifford Will called the “renaissance of general relativity” and which has been a major focus of research at the MPIWG for several years. This reconfiguration was a multifaceted social and epistemological process: one element of this was a renewed focus on the potential empirical implications of general relativity, such as in the collapse of massive stars.
The notion that general relativity might play an essential role in the collapse process that occurs when stars have burnt out their nuclear fuel was first advanced in the late 1930s by the US-American theoretical physicist Robert Oppenheimer and his graduate student Hartland Snyder. Their calculations appeared to indicate that a sufficiently massive star would, in collapsing, ultimately reach a density so high and thus a gravitational field so strong that it would “close itself off” from the outside world.
This striking conclusion was re-evaluated in the “renaissance” period, and was indeed frequently doubted as arising from too idealized a model of the collapsing star. One of the prime critics was John Wheeler, whose group at Princeton formed one of the hubs of the renaissance. Stefano Furlan of the Research Group Final Theory Program has investigated how Wheeler was led to give up his stark opposition in the early 1960s, due in part to the emergence of a more detailed picture of collapse processes from computer simulations. This development was reinforced when, around the same time, radio-astronomical observations revealed the existence of very energetic sources at the centers of distant galaxies, the so-called quasars. The unprecedentedly high energies of these sources could only be explained through the gravitational collapse of supermassive objects. The apparent existence of such objects, for which Wheeler was soon to popularize the name “black holes,” led to an intensified theoretical investigation.
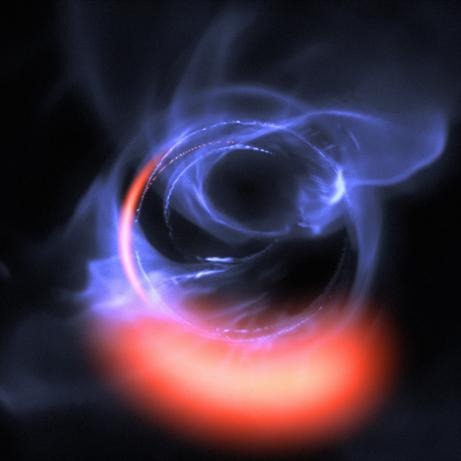
Simulation of gas orbiting at relativistic speed just outside the event horizon, the boundary of the Black Hole, beyond which nothing, including light, can escape. © ESO/Gravity Consortium/L. Calçada.
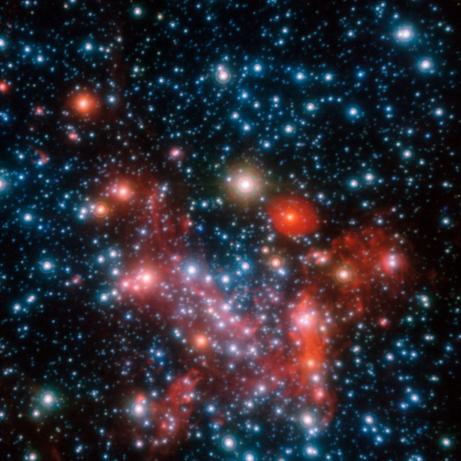
The central parts of our Galaxy, the Milky Way. © ESO/S. Gillessen et al.
The renewed investigation of gravitational collapse culminated in 1965 when 2020 Nobelist Roger Penrose showed that while black holes generically arise in the gravitational collapse of very massive stars, general relativity was unable to describe what happened at the singular point at the center of a black hole. This result established black holes as one of the frontiers of modern theoretical physics and as a central challenge in the search for a final theory of physics. Their role in this search continues to be investigated by the Research Group Final Theory Program.
The Search for Gravitational Waves and the Quest for Black Holes in the Max Planck Society
Black holes were protagonists of the first successful direct detection of gravitational waves in September 2015. The signals emitted from two black holes merging about 1.3 billion light-years, an extraordinary event opening a new observational window on the Universe, provided strong evidence for the existence of such extreme astrophysical objects.
The story of how scientists working at institutes of the Max Planck Society, Germany’s fundamental research organization, contributed crucially to all aspects of such detection, in particular to the unprecedented sensitivity of the Laser Interferometer Gravitational-Wave Observatory (LIGO) gravitational-wave interferometers, was studied in the context of the project on the History of Astronomy, Astrophysics, and Space Sciences in the Max Planck Society, as part of the wider program History of the Max Planck Society. This work has shown how the interplay between the renaissance of general relativity and the growing influence of relativistic astrophysics was instrumental in initiating gravitational-wave research at the Max Planck Institute for Astrophysics in Munich in 1970. The construction of increasingly large-scaled and innovative prototypes culminated in 1995, with the British-German gravitational-wave interferometer GEO600 and the foundation of the Albert Einstein Institute (AEI).
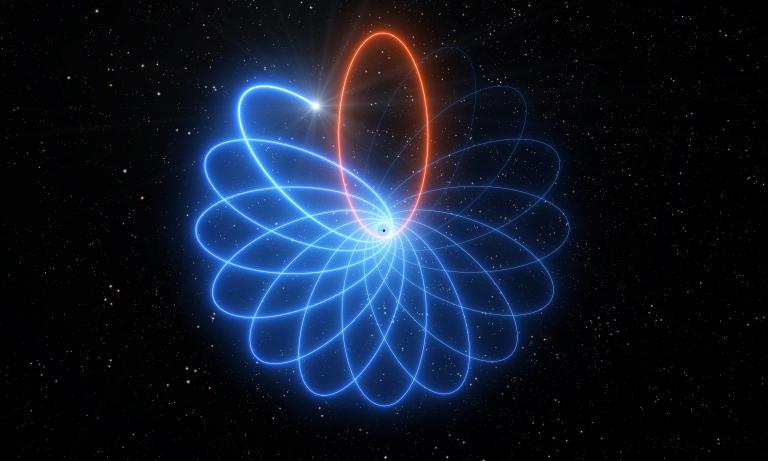
Illustration showing how the orbit of the star S2, moving very near the supermassive Black Hole at the center of the Milky Way, is not a closed ellipse, but moves forward creating a rosette shape, as predicted by Einstein’s theory of general relativity. © ESO/L. Calçada.
The project has also investigated how the long quest for the supermassive black hole at the center of our Galaxy became one of the central research themes at the Max Planck Institute for Extraterrestrial Physics in Garching, near Munich, with the appointment of Nobel Prize-winner Reinhard Genzel as director and head of the Infrared Astronomy Department. Based on the Max Planck Society’s unique technical expertise in instrument building, Genzel’s team entered the era of “observational black hole physics” with a dedicated long-term effort in infrared observations of radiation phenomena, gas dynamics, and especially of the proper motions and velocities of a group of stars tightly orbiting the supermassive black hole candidate at the center of our Galaxy.
Thanks to the advanced technologies of their very sensitive instruments, the international collaboration led by Genzel’s group was able to observe matter circling the “event horizon” the boundary of the black hole beyond which nothing, including light, can escape. And for the first time effects predicted by Einstein’s theory of General Relativity, such as the precession of the orbit and the redshift of the light of a star orbiting very close to the central mass, were observed in the strong gravitational field of a black hole of about 4 million times the mass of the Sun, whose existence was demonstrated by such observations “beyond any reasonable doubt.” The Nobel Prize in Physics 2020 has paid tribute to Roger Penrose, Andrea Ghez, and Reinhard Genzel, for their fundamental contribution in unveiling the “Milky Way’s darkest secret.”